Photobiomodulation Therapy (PBMT)
PBMT for pain/ inflammation reduction and tissue regeneration
Photobiomodulation (PBMT) therapy, also known as low-level laser therapy (LLLT), is a non-invasive healing light therapy that uses red and near infrared light to treat pain, reduce inflammation, and improve tissue repair. A lot of biological mechanisms has been proposed that are associated with promoting physiological change through PBMT. Near-infrared light in the 650-1000 nm wavelength range with a sufficient power density has been largely associated with PBMT. According to the first law of photobiology, for any photochemical reaction to take place within a biological tissue, the absorption bands of the chromophores present within the tissue must be able to absorb photons.
Cytochrome C oxidase (CCO) is the terminal enzyme (unit IV) in the electron transport chain situated in the outer mitochondrial membrane. During PBM, the mitochondrial chromophore CCO increases ROS generation, which in turn activates the PI3K/Akt pathway, while the effector enzyme, adenylyl cyclase, converts ATP to cyclic AMP (cAMP). cAMP could either activate pKA and RAS which further leads SIRT and ERK signaling to promote cell survival, inhibit inflammatory processes, and apoptosis. In the damaged cells, CCO, the protein that serves the purpose of end-point electron acceptor, becomes inhibited by non- covalent binding of nitric oxide (NO). When damaged cells are irradiated with low level NIR light, the photons in it will be absorbed by CCO leading to an increase in mitochondrial adenosine tri-phosphate (ATP) and release the nitric oxide which diffuses into the blood, increasing local blood flow and vasodilation.
An increase in mitochondrial membrane potential (MMP), ATP, cyclic adenosine monophosphate (cAMP), and NO is necessary for PBM to exert its effects. This is because CCO is activated in this process thereby catalyzing the reduction of oxygen to water. The activation of specific cellular networks requires NO as a signaling agent. PBM boosts NO production by cleaving metal compounds in cytochrome c and/or increasing cytochrome c levels. It is thought that PBM stimulates mitochondria-mediated cellular processes in damaged or dysfunctional tissue, leading to a broad variety of therapeutic effects.
Through continuous exposure of photons for a reasonable amount of time, a brief burst of reactive oxygen species (ROS) occurs in the mitochondria and activation of redox-sensitive genes, and related transcription factors including NF-κβ happens. The PBMT stimulates gene expression for cellular proliferation, migration, and the production of anti-inflammatory cytokines and growth factors. The role PBM plays in the mitochondria has brought it to the forefront as a potential therapeutic candidate in the treatment of neurodegenerative diseases, which are currently incurable due to their complex and multifaceted nature. Studies have also demonstrated the Neuroprotective efficacy of PBM in several models of neurodegenerative diseases.
The application of laser therapy favors angiogenesis, collagen synthesis, mitochondrial ATP production, cytokines and growth factors synthesis, in addition to inducing cell proliferation and differentiation. PBMT on diabetic wound healing using 810 nm (IR) wavelength and 650 nm (Red) light with a fluency of 60 J/cm2 could lead to a significant increase in fibro blast proliferation and collagen synthesis. Additionally, PBMT has anti-inflammatory, analgesic and bio-stimulating effects, acting mainly in the initial stages of tissue healing.
PBMT for weight loss
The effects of LLLT on adipocytes were attributed to formation of transitory micropores which were visualized on SEM (see Fig. 4) [24]. These pores were proposed to allow the release of intracellular lipids from adipocytes. Based on these data it was proposed that up to 99% of fat could be released from the adipocytes via application of 635 nm, 10 mW intensity LLLT for a period of 6 minutes [24]. One possible explanation might be that, increased ROS levels following LLLT initiate a process known as lipid peroxidation where ROS reacts with lipids found within the cellular membranes, and temporarily damages them by creating pores [22,57–59]. However, in an attempt to replicate Neira et al.’s data [24], Brown et al. [23] failed to visualize any transitory micropores. In another study, Medrado et al. investigated the action of different fluences (9 mW, 670 nm, 4, 8, 12, and 16 J/cm2 for 31, 62, 124, and 248 seconds, respectively) from a gallium–aluminum arsenide laser which was applied through the intact skin to the dorsal fat pad of rats. LLLT caused brown adipose fat droplets to coalesce and fuse, apparently transforming them into yellow fat but had only negligible effect on yellow fat itself [60]. Increased vascular proliferation, mitochondria, and congestion were evident findings in the laser irradiated brown fat. Considering most changes were restricted to the brown fatty tissue only and yellow tissue always preserved its appearance with no signs of lipolysis observed, results from this study were not in accordance with Niera et al.’s study. However, it is worthwhile to mention that experimental parameters used in these studies were not the same. Another possible mechanism of action for release of lipids was proposed to be through activation of the complement cascade which could cause induction of adipocyte apoptosis and subsequent release of lipids [29]. To investigate the complement activation theory, Caruso-Davis et al. [29] exposed differentiated human adipocytes to plasma. With and without irradiation there was noted to be no difference in complement induced lysis of adipocytes. Although no enzymatic assays were done to determine levels of complement within the plasma, the group concluded that laser does not activate complement. Lastly, unlike Niera et al.’s [60] findings, the external cell membrane preserved its normal appearance in electron microscopy, presenting no ruptures nor pores, in spite of the disposition of its fused fatty vacuoles, and no other signs of lipolysis were observed. An additional paper called into question the ability of red light (635 nm) to penetrate effectively below the skin surface and into the subdermal tissues [61]. In a supportive commentary Peter Fodor stated; “One could postulate that the presence of the black dots on SEM images on the surface of fat cells reported by Neira et al. could represent an artifact” [23].
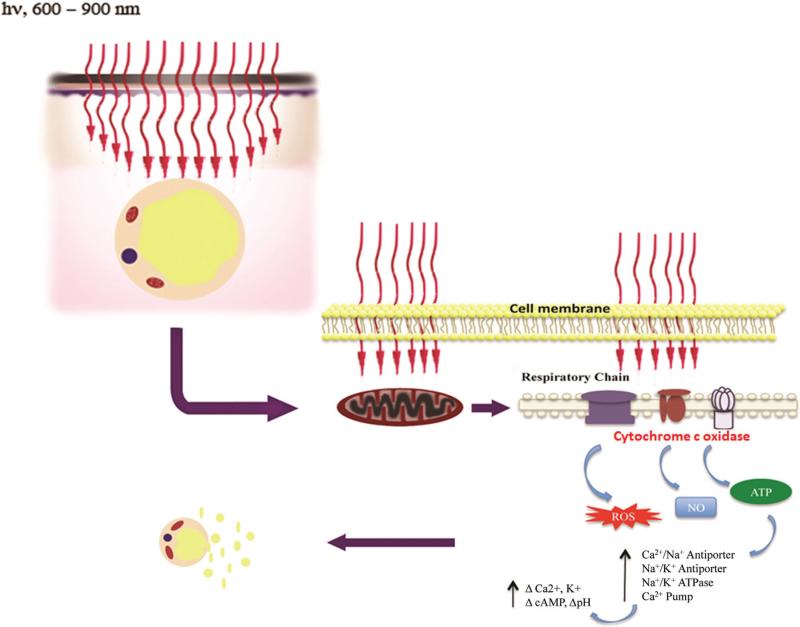
Photobiomodulation Therapy (BMT): What is it? How does it works? What can it do for you?
Juanita J Anders, PHD. Professor of Anatomy, Physiology and Genetics, Professor of Neuroscience, Uniformed Services University